This series will discuss the basic principles and methods of mechanical ventilation. Read the other entries here: (2), (3), (4), (5), (6)
The basics
The mechanical ventilator is a box that pushes air through a tube.
The first important concept to understand is this: ventilators work by positive pressure ventilation. Using a pump, they generate a higher pressure than the surrounding atmosphere and allow this to flow through a circuit (a sequence of plastic tubes terminating in an endotracheal tube within the trachea) into the patient’s lungs, making them inflate. Ventilators blow.
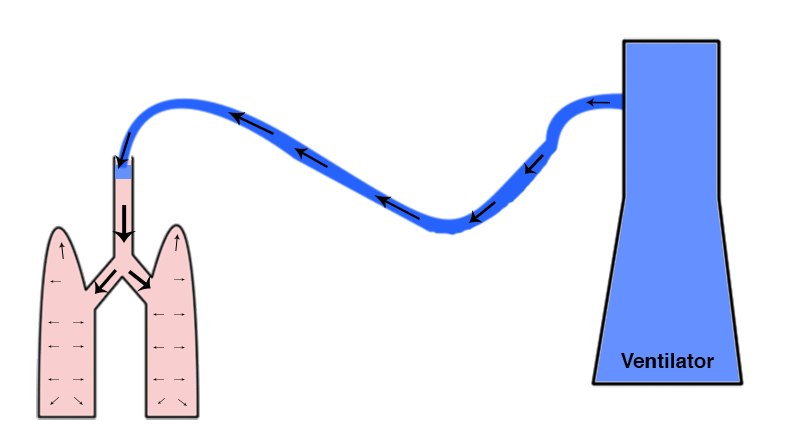
This is the opposite of our normal process of ventilation, which is negative pressure ventilation. Breathing spontaneously, we ordinarily drop our diaphragm and expand our chest wall, creating negative pressure in our thorax. This “sucks” our lungs outward, causing them to expand and transmit negative pressure into the airways. If those are open, air flows inward from the atmosphere. Off the vent, people don’t blow; they suck.
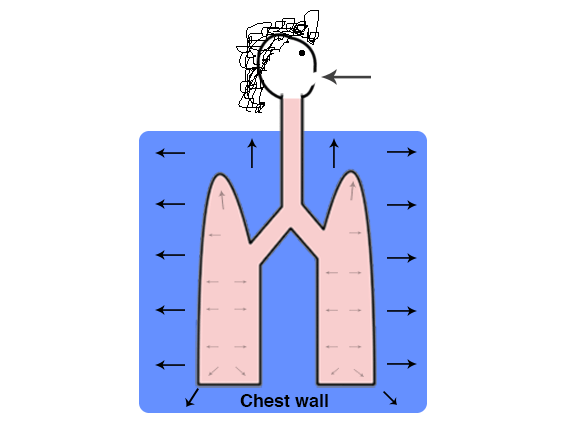
So the basic function of the ventilator is to create positive pressure, actively inflating the patient’s lungs. After this, exhalation is a passive process; we simply reduce or eliminate the positive pressure, allowing air to exit on its own as the lungs rebound back to their resting size. That’s all there is to it.
Anatomy of a breath: Pressure, flow, volume, and time
So mechanical ventilation is a recurrent cycling of positive pressure. Let’s establish the details of that pattern.
First we need to decide what each breath looks like. This will be defined by four interlocking characteristics:
- Volume: Measured in milliliters (ml, or the equivalent cubic centimeters—since 1 cc = 1 ml), this is the actual quantity of gas (the tidal volume) blown into the lungs and exhaled back with each breath.
- Pressure: Measured in centimeters of water (cm H2O), this is the pressure within the airway at any given time. When measured from the mouth of the ventilator during the inspiratory cycle, this is called the peak pressure.
- Flow: This is the amount of gas (the volume) blown through the ventilator per unit of time; thus it is the rate or speed at which gas is being delivered. The unit is liters per minute (LPM).
- And finally, an overarching variable of time, which is the duration of the inspiration in seconds—how long it takes to deliver the breath. The expiratory time is usually passive (the ventilator relaxes and allows the patient to exhale on their own) and therefore not “set,” although sometimes we wish it were faster.
Hopefully it makes sense that these variables are related: by changing one, you generally cause the others to change.
For example, let us take a single breath:
This breath had a total tidal volume of 500 ml. For most of the breath, the pressure in the airway was 20 cm H2O. The flow rate creating that pressure was a steady 50 LPM. Finally, since the flow was 50 liters per minute (aka .83 liters per second), and the volume was 500 ml (aka half a liter), you can guess that the inspiratory time was about .6 seconds. [erratum: numbers corrected 5/1/18 –ed.]
Now let’s twiddle some knobs:
Now our breath has a tidal volume of only 300 ml. Since the compliance of the lung (how “stretchy” it is, or the relationship of volume to pressure) is unchanged, the inspiratory pressure is now only 15 cm H2O. The flow rate in this particular mode is fixed, so it’s still 50 lpm. So by the same math, the inspiratory time is now only about .36 seconds.
Make sense? These variables are always intertwined, which is why most ventilators will graph them out for you: a continuous tracing of pressure, flow, and often volume, all against an X-axis of time.
A few more settings: Rate, FiO2, and PEEP
When will a breath actually be delivered? This can be triggered by various means. In modes capable of delivering full respiratory support, this will include setting a respiratory rate. More on this later.
The FiO2 is the concentration of oxygen in the delivered breath. You can deliver a certain volume of gas at a certain pressure, but the actual chemical admixture of gas you’re delivering is another matter. Modern ventilators are plugged into separate supplies of both medical air and pure oxygen, and an internal blender can create any mixture you desire from room air—21% oxygen (or .21, a notation with the same meaning)—up through 100% oxygen (1.0).
Finally, we can set a positive end-expiratory pressure, or PEEP. If mechanical ventilation produces a positive pressure within the airway to insufflate air, then relaxes during expiration to let the patient breath out, ordinarily at the end of expiration—when no pressure is being generated from either end—airway pressure would drop to zero. For many reasons, this is usually not desirable, so we have the ability to “hold” a certain amount of pressure in the circuit even between breaths. This can be set anywhere from 0 through a typical maximum of around 25 cm H2O.
That’s that
There you have it: the fundamental rules that determine how a ventilator will function. Next time, we’ll discuss the different modes that combine these principles and allow us to help patients breathe.